A task force to face the challenge
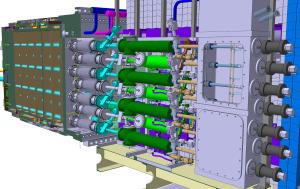
The international task force team is led by Michael Lehnen from ITER, who has worked in the field of disruptions for more than 10 years and coordinated the first disruption mitigation experiments at JET. The task force work is distributed over three groups. Experiments will aim on validating the ITER shattered pellet injection scheme (see more below) on today's tokamaks. The group is led by Nick Eidietis (General Atomics, USA) who is also the co-chair of the task force. He is a well-known expert in the field, coordinating the disruption mitigation work at the DIII-D tokamak. Technology, led by Uron Kruezi (ITER) and Nick Balshaw (CCFE, United Kingdom), has the focus on improving the technique itself and making it fit for the demanding environment in ITER. Theory & Modelling development is essential for the interpretation of the experiments and the extrapolation to ITER; Eric Nardon (CEA, France) and Akinobu Matsuyama (QST, Japan) will coordinate several work packages with a special eye on the physics of runaway electrons.
The concept that has been chosen for the ITER disruption mitigation system is based on a technology called shattered pellet injection, a technique developed at the Oak Ridge National Laboratory and pioneered at the DIII-D tokamak. Pellets enable the injection of massive quantities of neon and deuterium into the plasma in the form of solid ice. To ensure that the plasma can assimilate these quantities, the pellets are shattered into small pieces just before they enter the vacuum vessel.
The largest pellets for ITER disruption mitigation are larger than a wine cork, with a diameter of 28 mm. Despite this "enormous" size for a cryogenic pellet, several of these have to be fired at the same time to reach the required quantities to stop the worst-case runaway electron beam in ITER. A new layout of the ITER disruption mitigation system has been proposed that allows the injection of up to 32 pellets from equatorial ports at three different toroidal locations and three pellets from different upper ports. Although the equatorial port plugs are of substantial size, the designers are challenged when it comes to integration.
At its most recent meeting, the ITER Council approved the ITER Organization's strategy to tackle disruption mitigation and now the Task Force can get off the ground. To gain more confidence in whether the shattered pellet injection technique and especially the ITER disruption mitigation system design fulfils its aim, several tokamak experiments are planned and in preparation.
Next year will be a productive time with more and more tokamaks joining the shattered pellet injection community. Amongst the new members are JET, where commissioning of the shattered pellet injection system has already begun, and KSTAR, where the effect of dual pellet injection in a similar configuration to that in ITER can be studied.