Hotter than the sun
In the depths of the Sun where fusion reactions produce the energy that we perceive as light and heat, temperatures reach 15 million °C. In the centre of the ITER plasma temperatures will soar to between 150 and 300 million °C. How is such an environment possible? And what type of container will be host to an environment that is ten to twenty times hotter than the centre of the Sun?
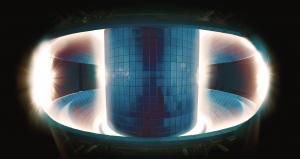
To answer these questions it is first necessary to ask ourselves about the nature of temperature. For a physicist, temperature is not only an indication of "cold" or "hot"; it also describes the energy of the particles that make up an object or a particular environment such as a plasma. The motion of particles such as nuclei, atoms and molecules (and the speed at which they travel) determine their energy.
For example, particles in ice (a cold solid) are tightly bound to one another and cannot move about at all. But as temperature rises, the agitation of the particles increases and the solid ice becomes water. At even higher temperatures, with particles moving at ever faster speeds, water becomes a gas (vapour).
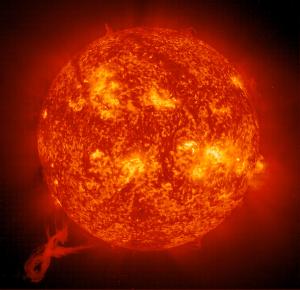
"Temperature" is not the same as "heat." Let's take the example of a fluorescent tube, which contains a gas such as neon, argon or mercury vapour that has been energized by an electrical discharge. Inside of the tube the temperature of the gas is elevated (on the order of 10,000 to 15,000 °C) but the tube can be touched without harm.
This paradox can be explained by the very low density of the gas within. Density is needed to transmit heat from an object to your skin (the higher the density, the more heat is transferred). An iron rod exposed to the Sun for an afternoon becomes scorching hot, whereas a piece of wood under the same conditions would never be too hot to touch due to its lower density.
Now let's return to ITER and to its plasma temperatures of 150 to 300 million °C. Plasmas are very tenuous environments, nearly one million times less dense than the air we breathe. The plasma particles are heated—that is, sped up—by different types of auxiliary heating methods until they are possessed of a formidable amount of energy. When they collide, the shock is such that the electromagnetic barrier that surrounds them is overcome, and the fusion of atoms can take place.
Despite its tenuousness, no physical cage can contain a plasma heated to temperatures of at least 150 million °C. If any part of the plasma were to come into contact with the surrounding wall, surface damage would result. But there would be even a graver consequence for ITER and for fusion: the plasma, despite the extraordinarily high temperatures at its core, would cool practically instantaneously in contact with the relatively cold vessel walls, stopping the fusion reactions immediately.
The solution physicists have found is to "imprison" the plasma inside a magnetic field—this is the concept of the "magnetic bottle" that was developed in the early 1950s and from which all present-day fusion machines derive. In a tokamak like ITER the hot plasma's charged particles are contained in the centre of the vacuum vessel by intense magnetic field and kept from physical contact with the interior walls of the machine.
Within the ITER machine, physicists and engineers are preparing to reproduce the physical reaction that, by fuelling the energy of the Sun, has maintained life on our planet for billions of years. In this very reaction is perhaps the solution for centuries of human development ahead—an energy source that is unlimited, clean and safe.
Follow these links to see videos of tokamak plasmas: JET (Europe), MAST (UK), JT-60 (Japan), and Tore Supra (CEA-Euratom).